Introduction
Hey there, science enthusiasts! 🌟 Let’s dive into the fascinating world of thermodynamics—a subject that’s not just for textbooks but essential in shaping the world around us. Whether it’s powering a factory, keeping your home cool, or creating the materials we rely on every day, thermodynamics is the secret sauce behind it all. And in chemical engineering? It’s an absolute game-changer! So, grab a coffee (or tea if that’s your vibe) and let’s break down the building blocks of this incredible field together.
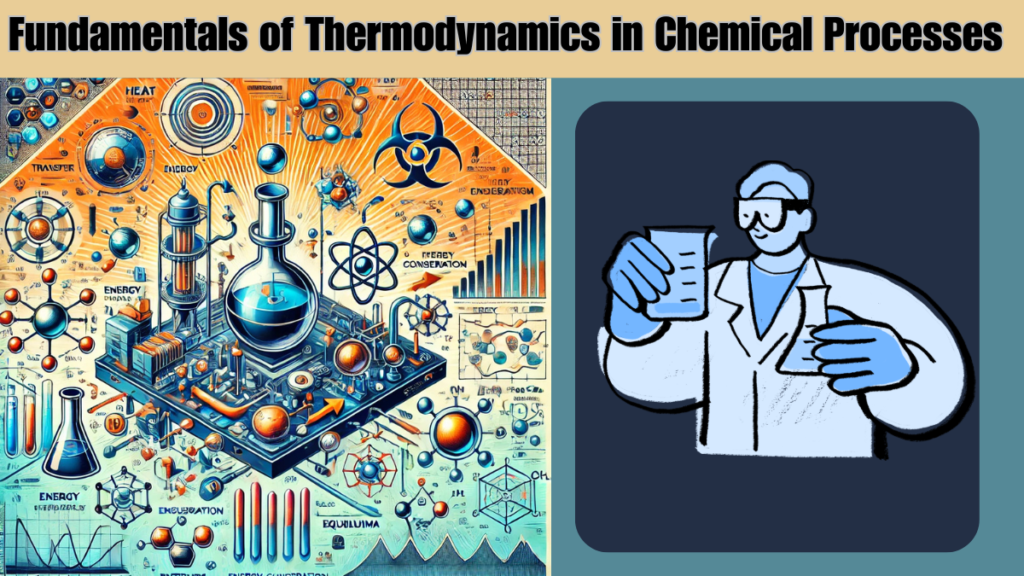
1. Introduction to Thermodynamics
So, what exactly is thermodynamics? Think of it as the ultimate study of energy—how it moves, transforms, and interacts with matter. In chemical engineering, it’s the foundation for designing processes that turn raw materials into useful products, like fuels, plastics, and even your favorite snacks.
Why is it so important? Imagine trying to bake a cake without knowing how an oven works. That’s what it’s like running a chemical plant without thermodynamics! It helps engineers predict how systems behave under different conditions, ensuring efficiency, safety, and sustainability. In short, thermodynamics is like the GPS guiding us through the complex maze of chemical processes.
2. Fundamental Concepts and Properties
Thermodynamic Properties: The Basics
Thermodynamics has its own language, and at its heart are properties like enthalpy, entropy, and Gibbs free energy. These are state functions, which means they depend only on the system’s current state—not how it got there. Some properties, like temperature and pressure, are intensive (independent of system size), while others, like volume and energy, are extensive (dependent on size).
These properties might sound technical, but they’re like characters in a story, each playing a role. Enthalpy helps us track heat during reactions, entropy explains why things tend to get messy (even in science!), and Gibbs free energy is the ultimate decision-maker for whether a reaction will happen.
Laws of Thermodynamics: The Ground Rules
Thermodynamics comes with its own rulebook. The Zeroth Law sets the stage with temperature and thermal equilibrium. The First Law, often called the conservation of energy, tells us energy can’t just disappear or magically appear—it’s always accounted for. And then there’s the Second Law, which gives us entropy, that cheeky measure of disorder that explains why your hot coffee cools down but never heats itself back up. The Third Law? It reminds us that absolute zero is the ultimate calm, where entropy reaches its minimum.
3. Energy, Heat, and Work in Chemical Processes
Forms of Energy
Energy comes in many flavors: internal energy, kinetic energy, and potential energy, just to name a few. In chemical processes, internal energy reigns supreme, as it’s tied to the bonds and movements of molecules. Add heat and work into the mix, and you’ve got a recipe for transformation.
Heat flows naturally from hot to cold, while work is energy transfer due to movement or force. Together, they’re the movers and shakers of thermodynamics, driving changes in chemical systems. Understanding this duo is key to designing systems that make the most of every joule of energy.
Thermodynamic Processes
Processes in thermodynamics are like unique stories, each with its own plot twist. In isothermal processes, temperature stays constant, while adiabatic processes avoid heat exchange altogether. Isobaric processes keep pressure steady, and isochoric ones lock in volume. These processes aren’t just academic—they’re critical for optimizing reactors, distillation columns, and heat exchangers in chemical plants.
4. Phase Behavior and Phase Equilibria
Equations of State
Every phase—solid, liquid, or gas—has its quirks, and equations of state like the Ideal Gas Law and van der Waals equation help us predict their behavior. These equations bridge the gap between theory and reality, especially when dealing with non-ideal systems where real gases and liquids defy simplicity.
Phase Diagrams and Critical Points
Ever seen a phase diagram? It’s like a map of matter, showing how temperature and pressure dictate whether a substance is solid, liquid, or gas. The triple point marks the magical condition where all three phases coexist, while the critical point is where liquid and gas become indistinguishable. These concepts are crucial for designing chemical separation processes, like distillation or crystallization.
5. Chemical Reaction Thermodynamics
Energy in Reactions
Every chemical reaction is an energy story. Some release energy (exothermic) while others need a little push (endothermic). The enthalpy of reaction tells us how much energy is involved, while heat of formation gives a snapshot of energy stored in compounds.
Gibbs Free Energy: The Ultimate Judge
Will a reaction happen? That’s where Gibbs free energy steps in. If it’s negative, the reaction is spontaneous (yay!), but if it’s positive, we need to intervene. Understanding Gibbs energy helps engineers design reactions that run efficiently and predict how temperature and pressure will affect yields.
6. Multi-component Systems and Mixing
In multi-component systems, we deal with mixtures instead of pure substances. Fugacity and activity replace simple pressure and concentration terms, helping us understand how components interact in non-ideal systems.
When substances mix, there’s a balance of energy and disorder. Mixing enthalpy measures the heat released or absorbed, while mixing entropy captures the increase in randomness. Together, they determine whether a mixture will form or separate, impacting processes like distillation, extraction, and emulsification.
7. Applications in Process Design and Optimization
Thermodynamics isn’t just theory—it’s the beating heart of process design. Take power plants, for example. Systems like the Rankine cycle (used in steam turbines) and the Brayton cycle (in gas turbines) rely on thermodynamic principles to convert heat into work.
In chemical plants, thermodynamics ensures separation processes, like distillation and absorption, run efficiently. It also drives innovations in heat exchangers, making sure energy is recycled and not wasted. By mastering thermodynamics, engineers can design processes that are both eco-friendly and cost-effective.
8. Advanced Topics in Thermodynamics
For those ready to dive deeper, statistical thermodynamics offers a molecular-level view of energy distribution. It connects the microscopic world of molecules with the macroscopic properties we observe.
Other exciting areas include exergy analysis, which pinpoints where energy losses occur, and computational thermodynamics, which uses simulations to model complex systems. These advanced tools are paving the way for breakthroughs in fields like nanotechnology and bioengineering.
9. Challenges and Future Directions
Thermodynamics has its limits, especially in highly complex systems like biochemical processes or nanostructures. Engineers face challenges in applying classical theories to these new frontiers.
But the future looks bright! With a focus on sustainability, thermodynamics is at the forefront of innovations in energy storage, renewable fuels, and carbon capture. It’s not just about understanding energy anymore—it’s about using it responsibly to shape a greener, smarter world.
10. Conclusion
Thermodynamics might seem like a dense topic, but it’s the backbone of chemical engineering and a guide to solving some of the world’s most pressing problems. From predicting chemical reactions to optimizing industrial processes, its principles are everywhere.
So, the next time you see steam rising from a factory or enjoy the convenience of a plastic product, remember the thermodynamic principles at work. Here’s to exploring the energy-packed world of thermodynamics—one equation, one process, one innovation at a time. 🌟