Introduction
Geotechnical engineering may sound a bit like rocket science, but it’s actually the incredibly cool branch of civil engineering that focuses on the behavior of earth materials. In simpler terms, it’s about understanding how soil, rocks, and other underground materials interact with structures we build on top of them. Geotechnical engineers are the unsung heroes behind everything from skyscrapers to bridges to tunnels. They study soil mechanics, analyze rock properties, and assess groundwater conditions, ensuring that any structure we build is safe, stable, and sustainable.
But why is this so important? Imagine building your dream house on unstable land or constructing a multi-story building on soil that can’t handle the weight. Yikes! This is where geotechnical engineers step in. They evaluate the ground conditions before construction even begins. Without their expertise, we wouldn’t have the confidence to walk into a building or drive across a bridge. These engineers are the ones who help us make sure the ground beneath us is solid, secure, and ready to support whatever is built above it.
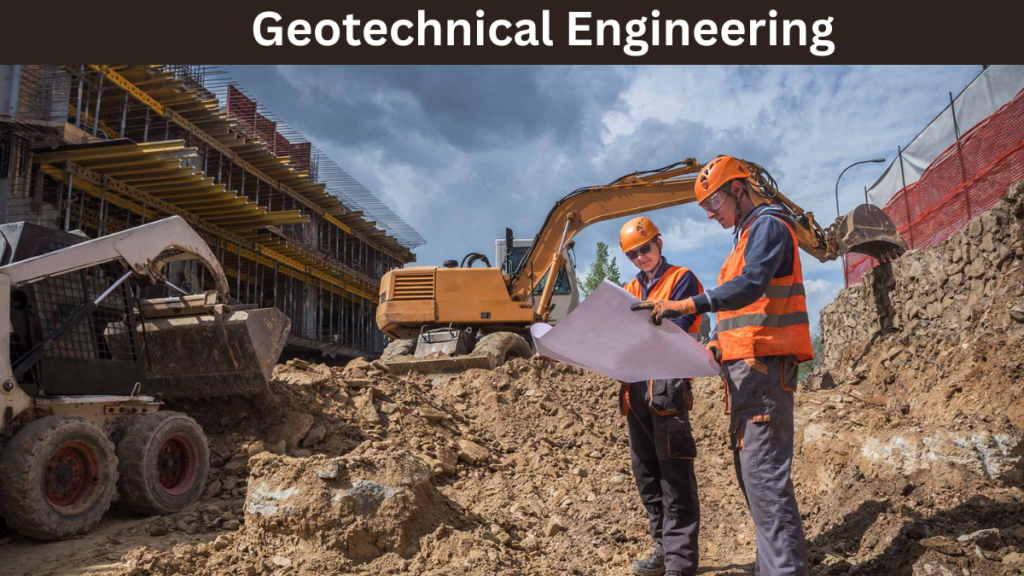
Soil Properties and Classification
One of the first things geotechnical engineers dive into is soil properties and classification. Just like people, soils have unique traits, and understanding them is key to figuring out how they will behave when subjected to stress. For instance, the texture and composition of soil can determine whether it’s going to be super stable or prone to shifting and sliding. Engineers look at grain size, compaction, plasticity, and moisture content to classify soils into categories such as sand, clay, or silt.
Soil classification helps engineers determine the right approach to foundation design and construction. Sand, for example, drains water really well and is typically less prone to liquefaction during earthquakes, while clay holds water and can expand or shrink depending on moisture. By knowing these characteristics, engineers can design foundations that suit each type of soil, whether it’s choosing shallow foundations for sandy soils or deep pile foundations for weaker, more compressible soils. Essentially, classifying soil is like solving a puzzle—it’s all about making sure each piece fits perfectly!
Site Investigation and Exploration
Now, let’s talk about one of the most exciting parts of geotechnical engineering: site investigation and exploration. Before any building or infrastructure can be constructed, engineers need to understand the ground they’re working with. They use a variety of tools and techniques to investigate soil conditions and collect data that will guide their designs. This is where those big drills, test pits, and complex instruments come in. They help engineers determine how deep the soil is, whether there are any hidden pockets of water, or if the ground is prone to shifting.
The information collected from site exploration doesn’t just sit in a notebook; it shapes every part of the construction process. For instance, during a site investigation, engineers might discover that the ground is softer than expected, prompting the need for a deeper foundation. They also use advanced field testing methods like the Standard Penetration Test (SPT) or Cone Penetration Test (CPT), which provide real-time data about soil strength and density. Think of it like giving the soil a physical exam to make sure it’s ready for construction—pretty neat, right?
Soil Mechanics Fundamentals
Soil mechanics is at the heart of geotechnical engineering, and it’s where the fun really begins! This branch is all about understanding how soil behaves when subjected to forces like weight, pressure, and moisture. For example, when a building is constructed, the weight of the structure applies stress to the soil beneath it, and understanding how the soil will react to this stress is crucial. The goal? Ensuring that the foundation won’t settle unevenly, crack, or sink into the earth. Engineers use different theories and equations to predict how soil will compress, shift, or shear under load.
Another fascinating concept in soil mechanics is consolidation, which refers to the process by which soil gradually compacts over time under sustained pressure. It’s kind of like how a sponge squeezes out water when you press down on it. If soil consolidation isn’t properly accounted for, it can lead to structural issues down the road. Soil shear strength is another key factor, as it determines how resistant soil is to sliding or failure under force. Engineers apply principles from soil mechanics to ensure that the foundation of any structure remains firm, stable, and safe—even under extreme conditions.
Foundation Engineering
Foundation engineering is one of the most vital aspects of geotechnical engineering because it directly impacts the stability of structures. Foundations act as the bridge between a building and the ground beneath it, transferring the weight of the structure to the soil in a safe and controlled manner. There are two main types of foundations: shallow and deep. Shallow foundations, such as spread footings and slab foundations, are used when the soil near the surface is stable enough to support the load. Deep foundations, like piles and caissons, are used when the surface soil isn’t strong enough to bear the weight, and the engineer needs to dig deeper to find more solid ground.
But designing foundations isn’t just about picking the right type; it also involves calculating things like bearing capacity, settlement, and the distribution of loads. Bearing capacity refers to the maximum weight that the soil can handle without failing, while settlement is the amount of sinking or shifting that happens over time. A good foundation should keep settlement to a minimum to avoid cracking or tilting the structure. For deep foundations, engineers must assess the type of soil at different depths to ensure the pile or caisson will anchor safely into solid ground. It’s a delicate balance between nature and design, but when done right, it’s a beautiful thing.
Slope Stability and Landslides
Geotechnical engineers don’t just deal with what’s below the surface—they also focus on what’s above it, especially when it comes to slopes. Whether it’s a hillside, embankment, or even a coastal cliff, slopes are prone to instability. A sudden shift in soil or rock can trigger a landslide, causing serious damage to structures and roads. This is where the science of slope stability comes in, and engineers apply their knowledge of soil mechanics to assess the likelihood of failure. They analyze the type of material, moisture levels, and external factors like earthquakes to determine if a slope is stable enough for construction.
Preventing landslides and slope failure often involves a combination of natural and engineered solutions. For example, engineers may install retaining walls, use geosynthetics to stabilize the soil, or even plant vegetation to reduce erosion. Sometimes, slopes can be regraded to improve stability, or drainage systems can be put in place to prevent water from accumulating and weakening the soil. It’s a dynamic and creative aspect of geotechnical engineering that keeps everything secure and safe, even in the face of Mother Nature’s challenges.
Retaining Structures
If you’ve ever driven by a hilly road or seen a building perched on a slope, chances are you’ve come across a retaining wall. These structures are essential in geotechnical engineering, as they help hold back soil and prevent erosion or landslides. Retaining walls are designed to resist lateral forces from the soil behind them and keep the ground in place, even under heavy pressure. Whether they’re made of concrete, stone, or even wood, these walls serve an essential role in maintaining the integrity of slopes and preventing soil movement.
There are different types of retaining walls, including gravity walls, cantilever walls, and anchored walls. Gravity walls rely on their weight to resist the pressure of the soil, while cantilever walls use a counteracting base to hold back the earth. Anchored walls, on the other hand, are secured with cables or anchors that go deep into the soil, providing extra strength in cases where the ground is particularly unstable. Retaining walls are designed based on the type of soil, the load from the structure, and the environmental conditions, ensuring that they stand the test of time and keep everything in place.
Earth Pressure and Lateral Soil Resistance
When engineers design retaining walls, they also have to think about the earth pressure pushing against them. Imagine trying to hold back a pile of sand—it’s going to exert a force on whatever it’s leaning against. The same thing happens with soil. The pressure exerted by the soil depends on factors like the type of soil, its moisture content, and the angle of the slope. Understanding earth pressure is vital to ensuring that retaining walls can withstand the forces acting upon them without collapsing. Engineers use a combination of theories, like Rankine and Coulomb’s Earth Pressure Theories, to calculate these forces and design walls that will stand strong.
Another key aspect in retaining wall design is lateral soil resistance, which refers to the soil’s ability to resist the movement of the wall itself. It’s all about balance: the soil needs to push against the wall enough to keep it in place, but not so much that it causes the wall to fail. Engineers use various equations to predict how much resistance the soil will provide and what kind of reinforcement is needed to maintain stability. It’s a delicate dance between nature and engineering that ensures everything stays safe and secure.
Soil Dynamics and Earthquake Engineering
Geotechnical engineering plays a crucial role in earthquake engineering, especially when it comes to soil dynamics. During an earthquake, the ground shakes and moves, causing soils to behave in ways they don’t under normal conditions. For instance, loose, saturated soils can undergo a process called liquefaction, where they temporarily lose their strength and act like a liquid. This can lead to buildings sinking or tilting. Engineers need to understand the soil’s behavior during an earthquake to design foundations that can withstand seismic forces.
To mitigate the effects of earthquakes, engineers perform seismic site response analysis, which involves studying how seismic waves will propagate through the soil and how they’ll impact structures. Depending on the soil type and density, some areas may experience stronger shaking than others. Engineers use this data to design foundations and structures that can absorb or resist seismic forces, reducing the risk of damage during an earthquake. By applying advanced